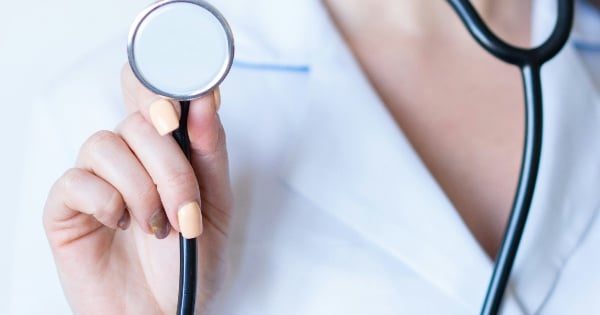

Dr. Steven Quay, M.D., Ph.D., founder of Atossa Therapeutics, discussed the cancer risk of CT scans, responding to a modelling study claiming that up to 5% of U.S. cancer cases may stem from CT radiation. He emphasized the need for scientific nuance, noting the body’s natural DNA repair mechanisms and differentiating high-dose from low-dose risks. He highlighted MRI as a safer alternative, although costlier and slower. Quay criticized the overuse of CT scans—especially in children—and the legal pressures that drive excessive diagnostics. He explored AI’s potential to enhance low-dose imaging and praised hybrid technologies like PET/MRI for advancing precision medicine while calling for better science communication and a return to scientist-as-adviser roles.
Scott Douglas Jacobsen: Today, we’re here with Dr. Steven Quay, M.D., Ph.D. He is a physician-scientist and the founder of Seattle-based Atossa Therapeutics Inc. (NASDAQ: ATOS), a clinical-stage biopharmaceutical company developing novel therapeutics for breast cancer and other conditions. He holds an M.D. and Ph.D. from the University of Michigan, completed a postdoctoral fellowship at MIT with Nobel Laureate Har Gobind Khorana, and served on the Stanford University School of Medicine faculty. He is the author of over 300 scientific publications, holds 94 U.S. patents, and invented seven FDA-approved pharmaceuticals. His current focus is on preventing the approximately two million annual breast cancer cases worldwide.
Thank you very much for joining today—I appreciate it. So, how credible is the estimate—based on the recent NPR reporting—stating that CT scans could cause cancer in up to five percent of annual cancer cases in the United States?
Dr. Steven Quay: I studied the paper carefully and did some background research. It’s a modelling study that does not track individual cancer cases directly but makes predictions.
The cancer risk from radiation exposure in this context is partly derived from long-term studies of the Japanese population that survived the atomic bombings of Hiroshima and Nagasaki. These individuals have been monitored for decades, providing valuable epidemiological data on radiation-induced cancer.
The fundamental issue with radiation-induced cancer is that while high doses are known to be associated with increased cancer risk, it’s less clear how risk behaves at lower doses. The question is: Is there a threshold dose below which radiation does not cause cancer? Or does any amount of radiation carry some risk, no matter how small?
One argument against the “no safe dose” assumption is the existence of over 30 DNA repair enzymes in humans. These enzymes evolved to repair DNA damage caused by various environmental sources, including natural background radiation. If evolution favoured the development of such robust repair mechanisms, it suggests that our bodies can tolerate—and effectively repair—low levels of radiation exposure without leading to cancer.
So, I believe there is a threshold dose below which radiation damage is fully repaired, and thus, the risk of cancer is negligible. But again, this remains an open scientific debate.
Let me pause here—if I’m not careful, I could go on at length. But there’s also the point that we’re all exposed to background radiation from multiple sources—cosmic rays, radon gas, naturally radioactive materials in the Earth, even certain foods—and CT scans need to be considered in that broader context.
It’s a far more nuanced issue than the modelling study or some headlines suggest. I am concerned that alarmist interpretations discourage patients from undergoing a CT scan when it’s medically warranted. That could ultimately do more harm than good.
That’s a high-level overview. We can certainly dive deeper into the specifics.
Jacobsen: How does all of that get translated through the filter of journalism and media—even some of the most respected outlets, like public radio and NPR? How much fidelity is lost in that process?
Quay: I read the NPR interview with the paper’s authors. And, unfortunately, it missed an opportunity. It would have been a great moment to frame it as a scientific debate—because I think that’s important.
As a side note, I believe the public—both in the United States and globally—has a fundamental misconception about science, and in some ways, scientists have misrepresented science. It’s almost a bilateral obfuscation. But people must see how scientists think, talk, and work.
This idea of the “shroud of scientific truth,” so to speak, was overplayed massively during the COVID-19 pandemic, leading to significant public misunderstanding and even distrust of science. So, we’re not in a good place right now.
Getting back to genuine science education and communication would be immensely helpful.
Jacobsen: What methodology—or what assumptions—underlie the projections in that study? Specifically, the claim that up to five percent of annual cancer cases in the U.S. are linked to CT scans?
Quay: Right. So, about 93 million CT scans are performed annually in the U.S. The researchers used cancer incidence data from populations exposed to radiation—particularly the Japanese survivors of the atomic bombings—where cancer outcomes have been studied over decades.
They applied that data to organ-specific radiation doses seen in CT scans. Then, they scaled those numbers based on usage patterns to project how many future cancers might arise. Essentially, it’s a numerical extrapolation.
We know pretty precisely how much radiation a CT scan delivers, and we have historical data on radiation exposure levels from survivors of Hiroshima and Nagasaki who were far enough from the blast to survive the initial explosion but still received significant radiation. By comparing those levels, the researchers created a risk model.
But here’s the challenge: CT doses are extremely low, so the absolute risk is also very low. This brings us back to a key question in radiobiology—does the risk line go through zero, or is there a threshold dose below which damage can be fully repaired?
We know that X-rays cause DNA damage. The question is whether that damage is repairable—and if so, whether the body’s mechanisms are sufficient to prevent cancer development at low doses. This paper is a projection based purely on modelling and assumptions. In my view, it doesn’t meet the clinical trial criteria or even a robust epidemiological study.
Jacobsen: How do you weigh the diagnostic value of CT scans against the long-term carcinogenic risks?
Quay: That’s a great question—and one I’ve been involved with for over 15 years out of a scientific career spanning four or five decades.
And full disclosure here—so there’s no perceived bias—I invented the contrast agent used in MRI imaging.
MRI is a very different modality from CT. MRI machines and scans are more expensive, so they’re used less frequently across large populations. However, MRI uses radiofrequency energy, not ionizing radiation like CT does.
As far as we can tell—and we’ve studied it for 30 to 40 years—MRI produces no long-term biological effects. So, while CT is a powerful and valuable diagnostic tool, especially in emergency or trauma settings, there are situations where alternatives like MRI can and should be considered, particularly when long-term radiation exposure is a concern.
So, MRI is an alternative to CT scanning. However, before MRI was introduced in the early 1980s, CT was already being developed and implemented. It’s particularly effective for imaging bones.
With CT, X-rays are passed through the body and absorbed at different levels depending on the composition of the tissue they pass through. Think of it like a photographic film—though CT doesn’t use literal film, the concept is similar. Denser materials stop more X-rays from reaching the detector.
Materials with a higher atomic number—those farther down and to the right on the periodic table—absorb more radiation. Most soft tissues in the body are composed of elements like carbon, hydrogen, and nitrogen, which do not absorb X-rays strongly. On the other hand, bones contain calcium and phosphate, which do. So, CT is excellent for evaluating bone and detecting bony lesions.
It’s not as suitable for soft tissue imaging. For example, the liver appears relatively homogenous on CT, whereas MRI can reveal detailed structures such as blood vessels and internal segmentation.
That said, CT remains the workhorse of radiology because it’s significantly less expensive—perhaps a fifth or a quarter of the cost of an MRI scan. As a result, many studies are conducted using CT.
I want to make a distinction here. The paper aggregates all age groups and all CT scans and projects approximately 100,000 cancers annually based on that combined dataset. That’s quite different from taking a more nuanced view—acknowledging that there are specific populations where CT scanning measurably increases cancer risk.
This is particularly true for pediatric populations. Children with cancer or other chronic conditions may require multiple CT scans over time. The concern is not just the repeated exposure within a short time frame but also the fact that they have a longer lifespan ahead of them in which a radiation-induced mutation might manifest as cancer.
Studies show there is about a threefold increase in brain tumours and leukemias among children who undergo multiple CT scans. That finding is very credible and well-supported.
However, the paper we’re discussing looks broadly at all diagnostic scans—many of which may be one-time exposures in adults—and draws population-wide conclusions.
Jacobsen: Are particular populations at greater risk from diagnostic radiation exposure? You already alluded to one group in your last response.
Quay: Yes, exactly—I may have gotten ahead of your question there. Children are more susceptible to the effects of CT radiation. But there’s another population I want to highlight: young girls, especially when it comes to chest X-rays.
The breast is unique in that it is not fully developed at birth. Breast tissue forms during puberty and originates from a minimal number of precursor cells—likely just a few thousand in the nipple region of a preadolescent girl. If radiation hits just one of those early cells, there’s a higher likelihood it could lead to breast cancer later on.
And I can share a personal story here. My daughter grew up in Seattle. At one point, she had a mild asthma episode, and we took her to Seattle Children’s Hospital for observation overnight.
Now, I’m an M.D., Ph.D., and my wife is a Ph.D. cancer biologist—she’s discovered major cell lines used in cancer research. Between the two of us, we’re highly trained and observant parents. We paid very close attention to decisions involving radiation exposure, especially for our daughter, precisely because of risks like the one I just described.
But the hospital was determined to do a chest X-ray because they thought our daughter might have swallowed a spoon—or something like that. But she had asthma, and it was affecting both sides of the lungs.
Now, if a child swallows a spoon or any foreign object, it would lodge in one bronchus or the other—not both. So, in that case, you’d expect asymmetrical symptoms: one lung would show signs of obstruction, and the other would be normal. This was a fundamental differential diagnosis. There were simple, non-radiative ways to confirm that it wasn’t a swallowed object.
We ended up taking her out of the hospital because we were afraid they might X-ray her in the middle of the night against our will.
There’s a phenomenon in medicine where we reflexively rely on imaging—especially X-rays—without always considering whether it’s necessary. I’ve taught many medical students, and one of the most critical questions they struggle to answer is this:
Let’s say we run this test. If the result is A, what will you do differently with the patient? If the result is B, what will you do differently? If the answer is: “Nothing will change,” then you should not do the test. The only valid reason to conduct a test is if it helps triage the patient into a different care pathway.
Jacobsen: Has medicine become overly reliant on CT imaging in diagnostic protocols?
Quay: Yes, I believe it has. And that speaks to how we’re training our medical students and clinicians.
As a medical student, I know the diagnostic process differed before CT and MRI. There was a guiding principle: imagine you’re in your office, with a long hallway leading to your door. The patient is walking down that hallway toward you. Your goal is to make the diagnosis before they reach the chair in front of your desk.
The point of that exercise is not to be showy or clever—it’s to emphasize that clinical observation, listening to the patient, and reasoned deduction are powerful diagnostic tools. In many cases, those skills are enough to arrive at a likely diagnosis or at least to rule out the most dangerous possibilities.
Unfortunately, in modern practice, we’ve become too quick to default to a battery of blood tests or a CT scan before fully engaging in patient dialogue and physical examination.
We could eliminate up to a third of CT scans currently performed I believe. And if you accept the modelling studies that suggest CT scans cause cancer, then eliminating a third of those scans would also prevent a substantial number of future cancer cases.
That would be a significant public health improvement that doesn’t require new technology, just better clinical decision-making.
The flip side of the conversation around unnecessary scans is the legal pressure physicians face. Some will argue, “If I misdiagnose something—even if I did everything right and simply chose not to order a CT scan—I could still get sued for malpractice.”
The legal environment may be contributing to overuse. Lawyers in the malpractice industry are likely responsible for increasing the number of diagnostic tests ordered. Because once you’ve done everything—X-rays, CT scans, labs—you can say, “I followed protocol. I did everything I could.” That’s more defensible in court than saying you didn’t run a particular test that, in retrospect, might have revealed a diagnosis.
Jacobsen: But those arguments, while dramatic in a courtroom—especially in American courtroom drama—often don’t reflect how diagnostics work in real clinical settings. Outside the legal concerns, what about reducing the cost of alternative scans that carry less long-term risk?
Quay: There aren’t many options. We’re working with three main technologies:
- X-ray/CT, which uses ionizing radiation;
- MRI, which uses radiofrequency and magnetic fields, and
- Ultrasound, which uses sound waves.
Each has its pros and cons. Ultrasound, for example, has limitations—it can’t penetrate bone, so you can’t use it effectively inside the chest or skull. It can scan between the ribs but not behind them. Dense materials block sound.
However, ultrasound can be beneficial for areas like the breast. There’s a condition called dense breast tissue, where the background tissue on a mammogram makes it challenging to detect cancer. In such cases, ultrasound can make the tumours more visible—they can practically “scream out” on the scan.
The issue with ultrasound is that it’s operator-dependent. The accuracy of the scan often depends on the person holding the probe. I’ve been involved in clinical trials where thousands of ultrasound studies were conducted, and when you segregated the data by technician, it became clear: one nurse was exceptional, and the rest were average or below average. That’s a fixed limitation with ultrasound.
Again, we’re mainly working with X-ray/CT, ultrasound, and MRI, which are your core modalities.
Jacobsen: What about AI integration? Is it relevant here?
Say the cost of all scans goes down—CT scans remain a third or a fifth of the cost of an MRI, but the absolute price falls to where CT used to be. Would that shift medical practice?
Quay: I think so, yes. Most people—patients and physicians—would prefer an MRI if it were cost-competitive.
But there’s a nuance to that. Time.
A CT scan can be completed in seconds. An MRI, on the other hand, can take 10 to 20 minutes, sometimes longer. That difference matters in emergency settings or even in busy hospital workflows.
When MRI machines were being priced out, we used to say that the cost—factoring in purchase, installation, staffing, and maintenance—was $20 to $40 per minute of scan time. That’s a serious economic factor.
So, even if scan prices come down, those time and infrastructure costs still weigh into clinical decision-making. But yes, if MRI becomes affordable and scalable, we’d see a shift in practice patterns toward its use.
There’s also the cost factor to consider. When you purchase an MRI machine—what we call “the magnet”—you usually take out a bank loan. You’re paying off that loan, and there’s pressure to maximize throughput.
That introduces a real-world constraint: How many patients can you scan daily? How efficiently can you get them in and out of the machine? These questions directly impact the economics of running an imaging center.
The underlying technology is nuclear magnetic resonance. When MRI machines were first introduced, they were called NMR machines. But that name scared people—”nuclear” anything tends to do that—and there was even a joke at the time: NMR stood for “No More Radiologists” because the machines produced such vivid images that some thought you no longer needed a radiologist to interpret them.
Jacobsen: We discussed the lack of many players or modalities in the imaging space. But in terms of reducing cumulative radiation exposure, what alternatives can be employed? For example, if someone needs multiple scans, could we alternate between CT and MRI, depending on availability, to reduce that cumulative risk—mainly if future, large-scale clinical trials support these cancer projections?
Quay: That’s a thoughtful idea, Scott. Let’s step back for a second and talk about why we imagine in the first place. Medical imaging generally serves two primary functions:
- Diagnosis – This is typically a one-time event. You use imaging—like mammography, for instance—to detect something, and once the diagnosis is confirmed, you move on to treatment.
- Therapy monitoring involves repeated imaging, usually to assess the effectiveness of a cancer treatment. Physicians track tumour shrinkage or growth over time using follow-up scans for inoperable tumours treated with radiation or chemotherapy.
That’s where the cumulative exposure comes in—and, notably, where the threefold increase in brain tumours and leukemias in children has been documented. These children often undergo multiple scans to monitor ongoing conditions.
Alternating between CT and MRI is creative—you’re the first person to suggest that to me. But in practical terms, it might be challenging to implement. The workflows, protocols, and data outputs are quite different. Clinicians must harmonize the imaging protocols and interpretation standards between the two modalities.
Jacobsen: How about combining CT and MRI datasets—and then using AI to integrate them for a more comprehensive image?
Quay: That is already happening, and it’s a fascinating frontier.
There’s a lot of AI innovation in imaging, especially in high-volume screening areas. Take Pap smears, for example. You collect cells from the cervix, place them under a microscope, and look for cancerous or pre-cancerous changes.
AI is now used to pre-scan those slides. It highlights areas of interest and flags them for the pathologist. A single slide may have 40,000 or 50,000 cells, but AI might identify a cluster of 15 cells that require close examination. This helps the doctor focus attention where it matters most.
The FDA has approved software that precisely guides the examiner from spot to spot with high precision.
Jacobsen: Is similar AI integration happening in mammography?
Quay: Yes. AI is also playing a growing role in mammogram interpretation. It assists radiologists by flagging suspicious regions, improving both speed and accuracy. It’s also beneficial in reducing false negatives and catching subtle findings that might be overlooked.
We’ve worked with the Karolinska Institute in Sweden on a really interesting mammography problem. One challenge with standard mammogram screening is called interval cancer. Most women receive mammograms every two years and they are both normal.
But there’s a small percentage of high-risk women who develop cancer between those scheduled screenings. That’s called an interval cancer—a cancer that arises in the interval between two mammograms. It’s incredibly frustrating, both for the women and the clinicians, because you’re doing everything by the book—getting regular scans—and yet cancer still appears in the interim.
So, we took a generative AI program and trained it on a massive dataset. We gave the AI 70,000 mammograms from women who did not develop interval cancers within two years and 7,000 mammograms from women who did. Then we asked the AI:
“Figure out what differentiates these two groups the greatest. Identify whatever diagnostic indicators you can find.”
The system ran continuously, 24/7, for a long time. When it was done, the results were impressive. While we don’t fully understand all the features the AI uses—it’s not always explainable—it was able to separate the two groups with about 90% accuracy. In other words, the algorithm can predict which women will likely develop interval cancer within the next two years.
We’re now using those findings to prepare to launch a prevention strategy using a drug I invented called Endoxifen. It blocks estrogen activity in the breast, quieting cellular behaviour in estrogen-sensitive tissues.
Estrogen is a wonderful hormone—it’s essential to what makes women—but it’s also a potent growth factor in tissues like the breast, uterus, and ovaries, which is why it’s linked to cancer development in those areas.
So that’s a case where AI has had a tangible, clinical impact. To put it into perspective, there are 39 million mammograms performed annually in the U.S., and about 10 million women have dense breast tissue. Within that group, we estimate there are 2 million women at elevated risk who could benefit from proactive treatment to prevent breast cancer.
Preventing even a fraction of the 250,000 breast cancer cases per year would be a significant public health achievement—and AI is helping us get there.
Jacobsen: When do you expect diminishing returns from a generative AI program like this? You’ve used a dataset of 70,000 non-cancer and 7,000 cancer cases. Would increasing the sample size to 350,000, for example, give you 95% or 99% predictive accuracy?
Quay: That’s an excellent question. The answer lies in the shape of the learning curve—and it’s an asymptote.
You do see diminishing returns as you increase the sample size. The early gains in accuracy are significant, but after a certain point, the improvements become more incremental despite larger and larger datasets.
Now, I have to switch hats—from scientist and physician to businessman—since I run a public company (Atossa Therapeutics, ticker symbol ATOS) focused on preventing breast cancer.
So here’s the business logic: If I can identify the top 10% of women most likely to develop breast cancer—even with just 90% predictive accuracy—and treat them with a pharmaceutical that has side effects no greater than a sugar pill, that’s a powerful intervention.
You’re getting substantial benefits with minimal downside. That makes much sense from a cost-effectiveness and public health perspective, even if you’re not hitting 99% predictive accuracy.
I’m probably comfortable with that risk-benefit ratio. Because I’m not going to harm someone who wouldn’t otherwise develop cancer—that is, the 10% of women who are flagged by the AI but wouldn’t go on to develop breast cancer.
If I can identify and treat the 90% of women who will develop cancer, even knowing that I won’t prevent all of them—because not all breast cancers are estrogen-driven—I still consider that a significant win.
When you dig into the biology, about 60% of breast cancers are estrogen-sensitive. So, if there are roughly 250,000 new breast cancer cases annually, and I can reduce that to 100,000 or 120,000 by using Endoxifen to prevent estrogen-driven instances, that means preventing well over 120,000 breast cancers a year.
That would be a big deal.
Jacobsen: I have two more questions. Are there any follow-ups to what you’ve said? For breast health, how do radiation concerns from imaging intersect with efforts in early detection?
Quay: That’s a critical point. I’ve already mentioned one high-risk population—preadolescent girls. To perform a chest X-ray on a girl in that age group, you need to have a powerful clinical justification. And parents need to ask the right questions:
- What are you expecting to see on this X-ray?
- If you see it, how will that change the treatment plan?
60% of chest X-rays in children wouldn’t hold up to that kind of scrutiny. And we should be asking those questions. There’s a reflex in medicine—without casting blame—especially in top-tier pediatric institutions, to follow set protocols.
For example, if a child presents with asthma, do A, B, C, and D, and include a chest X-ray. It’s part of a checklist, but it shouldn’t be. We need to move away from that kind of reflexive imaging.
So yes, adolescents and children are one group we should be particularly cautious about.
Mammograms are quite safe when done properly. We’ve reduced the X-ray dose in modern mammography by eight to tenfold compared to previous generations. So, women today receive only about 10% of the radiation dose used decades ago.
One of my criticisms of the study we’ve been discussing is that it relies on historical data from older, higher-dose X-rays. The field has advanced significantly since then.
Now that you’ve raised the topic, I have an idea I hadn’t fully considered before: AI might allow us to reduce radiation dose even further by enhancing the degraded image and reconstructing detail from lower-energy scans.
That’s a very promising avenue. I suspect the next generation of X-ray technology will follow suit, relying on AI-assisted reconstruction to allow for even lower radiation doses without sacrificing diagnostic quality.
And finally, we need to be highly cautious with radiation exposure in areas like the breast and the ovaries.
- The breast, especially during development, is susceptible to radiation.
- The ovaries are even more sensitive. A woman is born with all the 40,000 to 50,000 oocytes she will have in her lifetime, and any damage to those affects not just her health but potentially the next generation.
We use lead aprons during imaging to protect the reproductive organs when exposure is unavoidable.
When imaging the abdomen, it’s primarily soft tissue—few bones obstruct the view. In many cases, ultrasound is an excellent, non-radiative option for abdominal imaging.
You can use ultrasound to assess several abdominal issues. You can see if the appendix is enlarged, evaluate liver blood flow, check for splenic enlargement, or even detect a bowel obstruction. You can also get a sense of where the obstruction is. So yes, ultrasound has many diagnostic strengths—especially in soft tissue evaluation.
Now, when it comes to bone lesions, CT is still superior. By definition, CT imaging is better for bone than MRI because bones appear dark—almost black—on an MRI scan. That’s because bones don’t emit a strong signal in MRI; they’re primarily signal voids.
Jacobsen: That leads to something I mentioned in the previous question—what innovations might reduce reliance on radiation-based tools? Specifically, what if we reduce or degrade the radiation in an X-ray or CT scan and then use AI to reconstruct or enhance the image?
It reminded me of something Dr. Chen Wang presented recently—he discussed how AI can take a few pixels and predictively fill in a complete image using advanced algorithms. That kind of generative pixelation already works for entertainment and digital art. So why not apply it in clinical imaging?
Quay: That’s entirely possible, and I find it very exciting.
When you think about CTs or MRIs, you’re essentially dealing with 2D representations of 3D structures. These images are captured in cross-section, typically with about 1-millimetre in-plane resolution and about 1-centimetre thickness per slice.
So imagine these scans as a series of vertical columns—almost like looking through the body like a magician has sliced it into layers. Each image gives a thin, cross-sectional view of anatomy.
To clarify something you raised, we don’t want to take one high-resolution quarter of the image and ask AI to extrapolate the rest—that doesn’t work due to the anisotropic nature of human anatomy. In other words, knowing what’s on the right side of the body won’t let you accurately guess what’s on the left side. The human body isn’t perfectly symmetrical or predictable that way.
Instead, we’d want a low-resolution image of the whole organ or region and then use AI to enhance the resolution uniformly. That is feasible and very exciting. You’re getting diagnostic value from less data, which means less radiation in the case of X-rays or CT.
Mathematically, this is similar to the principles behind Pointillism in art. It’s like the work of Georges Seurat, the French artist who pioneered Pointillism. He painted entire scenes using tiny dots, and when you stand up close, all you see are those individual dots. But when you step back, your eye blends them into a coherent image.
Jacobsen: So you could imagine a future in which we capture only a partial-resolution image with low radiation and then use AI to “fill in the dots,” so to speak.
Like Seurat’s paintings, where the whole picture only emerges from the collection of points, a high-fidelity medical image might be reconstructed from limited data, enhancing safety and diagnostic power.
That approach—applying generative models from art and imaging science to clinical radiology—could be one of the most essential frontiers in medical imaging. And it’s not science fiction. It’s within reach.
Quay: I agree. My point is that we understand the mathematics behind how an image—when viewed at a distance—comes together to display anatomical detail. But when you zoom in to the pixel level, you can analyze how density transitions from one pixel to its neighbouring pixel occur. You see gradients and slopes in intensity values, and those transitions can be mathematically modelled.
AI can replicate those patterns with high fidelity by analyzing these local changes in density across neighbouring pixels. Thus, generative AI can enhance resolution in medical imaging very well.
It’s also true that the grayscale spectrum—the difference between black and white tones in a CT image—is directly tied to the X-ray dose. You have a curve: if the image is underexposed, it looks all white; if it’s overexposed, it looks all black. The ideal image sits in the middle of that S-shaped exposure curve—the “sweet spot.”
Most current CT systems aim to operate in that optimal range. But if we could reduce the dose—bringing us slightly down that curve toward lower radiation—we could then use AI to reconstruct or restore the detail that would otherwise be lost. That process would be quantifiable and demonstrable. You could calculate how much dose you can safely reduce and how much AI would need to compensate.
Jacobsen: That makes sense. Do you also need to calibrate the radiation based on someone’s body size—a taller person with a higher BMI and more bone, muscle, or fat tissue?
Quay: Absolutely. That’s a critical part of how diagnostic radiology works.
Before a full scan, a very low-dose calibration or scout scan measures the patient’s body habitus—tissue density and size. Based on that measurement, the system automatically adjusts the radiation dose to give the image enough penetration and contrast for diagnostic clarity while still using the lowest necessary dose.
Jacobsen: Let’s say you had fewer dots—or, in our case, fewer pixels. AI could fill in the missing information using statistically reasoned interpolation, much like Seurat’s work, where the viewer’s eye blends the dots into a coherent image.
This is very similar to image enhancement techniques used in social media applications today—except we’d be applying them to clinical diagnostics, not entertainment.
Quay: If you have large pixels and abrupt differences between them, the AI makes the pixels smaller and smooths the gradient between values. That’s mathematically straightforward. We can improve diagnostic quality from a clinical imaging standpoint while potentially reducing radiation exposure. That’s a win for patient safety and diagnostic precision.
With CT imaging, we’re not dealing with colour—just a grayscale. You’re looking at 256 shades of gray, more or less.
Jacobsen: Switching gears: in the broader field of radiology and cancer diagnostics, what are some changes you’ve seen over your career that, perhaps obvious to seasoned experts, have only recently been implemented or adopted systematically?
Quay: That’s a great question—and I think we’ve touched on some of them already.
The continual improvement in both CT and MRI has been remarkable. When I first invented gadolinium-based contrast agents for MRI, it used to take about 20 minutes to capture a single image of the heart. Now, we can generate real-time videos of the heart—20 frames per second—allowing clinicians to watch the heart pump in action.
Both spatial and temporal resolution have improved dramatically, and radiology has seen one of the most significant advances over the past few decades.
But I do want to make a cautious observation here. It’s always risky to declare that we’ve hit a plateau—like claiming civilization is at its peak, and everything after is declining. But in radiology, it does feel like we’ve entered a phase of incremental improvement rather than revolutionary change.
We haven’t been back to the Moon since I was young. So, just like in space exploration, radiology may be in a stable phase now. That’s not bad—it just means we’re refining what works rather than inventing entirely new modalities. The invention of MRI was worth one, maybe two Nobel Prizes because it was such a transformational technology. But I don’t think there’s another imaging modality on the horizon of that magnitude—at least not yet.
That said, there have been innovations in hybrid imaging. Take prostate cancer, for example—not diagnosis, but staging. In this context, a blending of modalities is happening—specifically, the integration of PET—Positron Emission Tomography—with CT or MRI. PET allows you to visualize metabolic activity in tissues using radioactive tracers.
PET uniquely uses physics.
Unlike traditional radiation, which radiates outward in all directions—like an expanding sphere—positron emission involves the release of two particles travelling in opposite directions, typically at 180 degrees to each other. The scanner is programmed to only record the simultaneous events, not single events, and so is a very sensitive method.
Because of that predictable behaviour, you can detect the origin point with remarkable precision. That makes PET incredibly useful for detecting areas of active metabolism, like cancer cells, and mapping them in 3D when combined with CT or MRI data.
One of the recent innovations is fused imaging, which combines anatomical imaging (like CT or MRI) with functional imaging (like PET). That hybrid approach gives you both structure and activity, and it’s already transforming cancer staging, particularly for prostate, lung, and certain lymphomas.
So, if you put a circular detector around a person who’s been given a radioactive tracer that emits positrons and configures the system only to detect coincident particles—that is, two particles hitting opposite sides of the detector simultaneously—you drastically reduce background radiation noise.
Background radiation is a real thing. We haven’t talked about it yet, but it’s essential. For instance:
- Cosmic radiation accounts for about 20% of the dose from a chest CT.
- The Earth is radioactive, contributing about a third of a typical CT dose.
- Even foods can be radioactive—bananas are a classic example due to their high potassium content. You can get a reading on a Geiger counter if you hold it near a banana.
- Radon gas—a naturally occurring radioactive gas—can seep from the ground into homes. In certain regions, homebuyers will do a radon test, and homes may be fitted with gas-impermeable barriers to block radon exposure.
Positron emission tomography (PET) has a very low background signal because it’s rare for cosmic rays or Earth-based radiation to produce two coincident particles 180 degrees apart. That’s why PET imaging is highly sensitive.
But here’s the trade-off: PET has very high sensitivity but poor spatial resolution.
If you’ve ever seen a standalone PET scan of a patient with prostate cancer or metastatic breast cancer, the images are crude. You can barely make out a human shape like a child’s stick figure drawing. You see vague outlines: maybe two legs, some glowing clusters. Not very detailed.
So here’s the innovation in prostate cancer staging:
Researchers took an antibody that binds specifically to prostate cancer cells, attached a PET-emitting radionuclide to that antibody, and then embedded the PET scanner inside an MRI machine.
Now, that’s expensive—General Electric loves it—but it’s powerful. You get the sensitivity of PET, down to just a tiny cluster of cancer cells, and the high-resolution anatomy of MRI. With that, a surgeon can say, “Here’s exactly how I can reach that tumour cluster,” or a radiation oncologist can say, “I know precisely how to target this with external beam therapy.”
These are powerful multimodal imaging tools. And again, this isn’t theoretical. It’s already happening. So, while I wouldn’t say we’re in the sunset of innovation in radiology, the field is grounded in physics—and physics doesn’t change easily. We’re working within the laws of nature.
Jacobsen: That reminds me of something Alexis Rockman—the artist behind the original Earth Day poster—once said. He’s done the activist circuit and is incredibly talented.
He whimsically but seriously said that climate change is a physics experiment. Whether we protest or not doesn’t change the underlying laws of physics. We either act or we don’t. Nature doesn’t care about ideology.
So, in the same way, your point about radiation is similar: physics is physics. I’d love to hear your thoughts on something James Randi used to talk about: the “woo” ideas people have about radiation versus the realities. What are some common misconceptions people have about radiation?
Quay: Yes, great question. And you’re right—just like with climate science, the laws of physics don’t care whether you believe in them. They operate regardless of opinion.
There are quite a few radiation misconceptions.
Many people think all radiation is harmful or that even small exposures are deadly. But that’s not true. We’re exposed to radiation constantly—from space, the Earth, and even inside our bodies. And we’ve evolved with mechanisms to handle low levels of radiation. The problem comes with high, repeated, or unnecessary exposures.
People also tend to conflate all types of radiation—like confusing non-ionizing radiation (such as from your phone or microwave) with ionizing radiation (like X-rays or gamma rays). They’re not remotely the same in terms of biological impact.
The key is to understand context, dose, and duration. Just as a small amount of radiation from a medical imaging scan can provide life-saving information, so too can we manage radiation risks intelligently without falling into paranoia or ignoring real hazards. The general public still significantly misunderstands radiation’s risks, applications, and how it works.
Take nuclear energy, for example. It is the most viable solution if we genuinely want to replace fossil fuels. You cannot fully replace fossil fuels with wind and solar alone. The reason is simple: they’re intermittent. The sun doesn’t always shine, and the wind doesn’t always blow. You need a reliable backup, and that’s where nuclear could come in.
Nuclear and fossil fuels—apart from the portability of fossil fuels but not nuclear—are functionally identical when it comes to producing constant, base-load power. However, due to early atomic plant designs that lacked sufficient safety measures and the catastrophic events that followed, public fear of nuclear energy skyrocketed and has not subsided.
As a result, building new nuclear plants has become politically and socially complex. Existing plants are being decommissioned, and they are unlikely to be recommissioned. That’s a huge missed opportunity. Today, we can engineer extraordinarily safe nuclear reactors, literally fail-proof. But irrational fear is standing in the way—fear rooted in radiation anxiety, not objective risk assessment.
The same principle applies to medical imaging—especially CT scans. People often overestimate the danger.
Here’s a simple comparison: if you fly over the polar routes—from Asia to North America or Asia to Europe—three times, the cosmic radiation you absorb is equivalent to one head CT scan.
If you trust that the greater than 30 DNA repair enzymes in your body are still functioning properly—no matter your age—then there’s no need to fear low-level radiation from a medically indicated CT scan. We are, quite literally, built to tolerate a reasonable amount of radiation.
Jacobsen: Last question—complete side note, but it comes up often in the news: How dangerous are cell phones?
Quay: That’s a common concern, and I’m glad you asked.
Cell phone networks transmit signals via radio frequency (RF) waves. As data demands increased over time, engineers had to increase the frequency bands to allow more users and data to pass through the same bandwidth—like widening a digital highway.
So, each generation of mobile technology—from 2G to 5G—uses higher frequencies to boost signal-carrying capacity.
The scientific literature shows that the human body has a limited biological response to these radiofrequency waves. There is no credible evidence that they cause cellular damage or increase cancer risk.
Particular frequencies do interact with the human body—the foundation of MRI. In an MRI machine, a powerful magnetic field aligns the hydrogen protons in your body (mainly from water). Then, a precisely tuned radiofrequency pulse is applied, causing the protons to flip their orientation. When the pulse is turned off, the protons return to their baseline at different rates depending on the tissue. That’s what gives you the contrast in an MRI image.
So yes—radiofrequency waves can interact with the body—but only at particular frequencies, controlled conditions, and much higher energy levels than cell phones emit.
Cell phones operate far below that threshold. They do not have the energy to disrupt electron orbitals or affect molecular bonds in human tissue. So, based on everything we know and all the best available data, cell phones are not dangerous in this context.
So, hydrogen has one electron, helium has two, carbon has six, and so on. Life as we know it is built on the interaction of electrons. That’s electron chemistry—not nuclear chemistry.
The nucleus is so powerful and tightly bound that you can’t alter it through everyday chemical processes. The only way to do nuclear chemistry is through nuclear reactions—think Oppenheimer and the atomic bomb. That’s an entirely different domain of physics.
But electron chemistry—yes, it may seem not very interesting, but it’s the foundation of all biological life. So, while radio frequencies from cell phones can theoretically cause resonance in the hydrogen protons in the nucleus of the water in your body, they don’t disrupt the nucleus or disrupt atomic structures. And as far as we know, they have no biological impact on those energy levels.
Jacobsen: Any final thoughts—not necessarily about radiology specifically—but about the NPR report or the study behind it?
Quay: Yes. It’s critically important for scientists to engage in public debates on issues of broad significance. And I want to emphasize this: science is never settled.
Now, gravity has never failed us—it’s always waiting. I joke with my kids, “Gravity is always there—it’s just waiting for you to slip.” But even gravity is still described by a theoretical model. And beyond such fundamentals, most areas of science are far more fluid.
The notion that science is fixed—a belief that emerged prominently during the COVID-19 pandemic—is simply incorrect. Science evolves through iteration, refinement, and continuous questioning.
Unfortunately, during the pandemic, scientists often crossed the line between being advisers and becoming policymakers. That’s not our role.
The scientist’s job is to say, “If you implement policy X, here are the likely outcomes—positive and negative.” For example, if you put masks on children still learning to speak, a scientist might advise: “Well, their language development may be impaired because children learn by mimicking facial expressions and mouth movements.” That’s a valid, science-based point.
However, the decision to mask or not should be made by elected policymakers, not scientists acting as de facto authorities. Unfortunately, we saw too much mission creep—science dictating rather than informing policy.
We need to restore that boundary. Scientists should provide insight, not edicts. And those making laws should be the people we vote for.
Right now, governance has become lopsided. Too many decisions are made by individuals we don’t vote for rather than those we elect. Society may need to reevaluate and recalibrate this.
But at its core, science remains humanity’s greatest invention. The scientific method—designing controlled experiments, isolating variables, forming hypotheses, testing them, and iterating—has given us almost everything in modern life, including, for example, the video call we’re on right now and the network that enables it across thousands of miles.
It is, truly, the hallmark of civilization.
And the next stage, our partnership with generative artificial intelligence is upon us.
We are now enter uncharted territory where, as AI approaches or even exceeds human intelligience, the master-slave relationship between humanity and technology may actually be shifting. That’s another debate we’ll need to have as a society.
Jacobsen: Dr. Quay, thank you very much for your time today. I appreciate your expertise and your willingness to explore many off-track but essential topics, as tends to happen in my interviews.
It was a pleasure to meet you.
Quay: Same here, Scott. Take care.
Jacobsen: Take care. Bye-bye.
—
Scott Douglas Jacobsen is the publisher of In-Sight Publishing (ISBN: 978-1-0692343) and Editor-in-Chief of In-Sight: Interviews (ISSN: 2369-6885). He writes for The Good Men Project, International Policy Digest (ISSN: 2332–9416), The Humanist (Print: ISSN 0018-7399; Online: ISSN 2163-3576), Basic Income Earth Network (UK Registered Charity 1177066), A Further Inquiry, and other media. He is a member in good standing of numerous media organizations.
***
If you believe in the work we are doing here at The Good Men Project and want a deeper connection with our community, please join us as a Premium Member today.
Premium Members get to view The Good Men Project with NO ADS. Need more info? A complete list of benefits is here.
—
Photo by Alexandr Podvalny on Unsplash
Disclaimer: This story is auto-aggregated by a computer program and has not been created or edited by healthlydays.
Publisher: Source link